Core Topics
Membrane processes can be employed for the separation of liquid, vapourous or gaseous mixtures. Compared to its conventional competitors, membrane processes are often advantageous due to their low energy consumption, simple process design and small footprint. Often membrane processes can be advantageously combined with other separation and reaction processes to hybrid processes. Applications of these processes can be found in the chemical and petro-chemical industries, in renewable and conventional energy technology, in biotechnology,
in environmental technology, in the food industry and in the pharmaceutical industry.
The department “Process Engineering” is concentrating on gas separation membrane processes with the focus on polymer based gas and vapour separation membranes. These membranes work according to the solution-diffusion principle.
This principle necessitates the application of a driving force across the thin, dense selective polymer layer of the membrane. The driving force causes a solution of the components present in the feed mixture into the polymer matrix and a subsequent diffusion of these components in their dissolved state to the permeate side of the membrane where they are desorbed.
The separation is caused by the different interactions of the various components of the feed mixture with the polymer of the selective layer(s) in the solution and diffusion steps.
Essential for successful implementation of research results into industrial applications is that the entire research and development chain is considered holistically. In the department of “Process Engineering” we work on the following:
- Membranes for
gas separation - Permeation performance of
gas separation membranes - Production of flat
sheet membranes - Membrane
modules - Pilot scale membrane
process characterisation - Modelling and
simulation - Membrane process
design
Applications of membrane processes
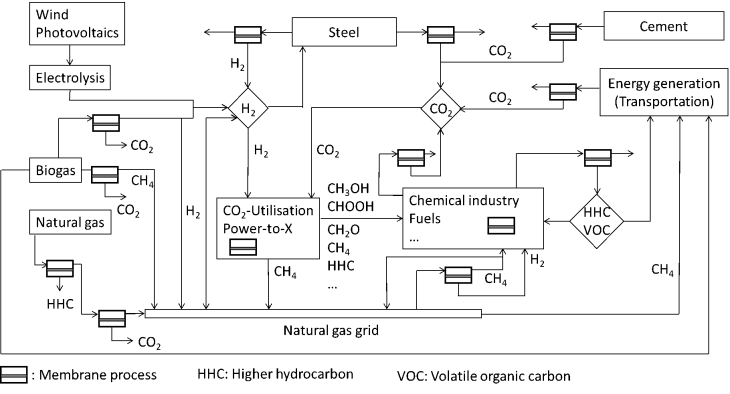
Image: Hereon/Torsten Brinkmann
Membranes for gas separation can be employed for numerous separation tasks. In the focus are carbon dioxide (CO2) and hydrogen (H2). Even for the current industrial society aiming at the avoidance of CO2 emissions, it will not be possible to live completely without point sources of CO2. Various Hereon membranes are designed for the separation of CO2 from different gas mixtures as flue gases, biogas, natural gas or process streams in the chemical industry.
The situation with H2 is similar. Here, the separation from process gases in the chemical industry is an important application as well, but also the separation of H2 from the natural gas grid in order to supply it for further usage with the required purity could be an important future application. Additionally, polymeric membranes were developed for the separation of higher hydrocarbons or water vapour from permanent gases.
The majority of the membranes are produced as thin film composite membranes. This allows for the production of large membrane areas using small amounts of high performance polymer. Often 100 mg of polymer are sufficient to prepare
1 m2 of membrane area. The stability of the membrane is provided by other, more cost efficient polymers. It is also possible to produce gas separation membranes according to the phase inversion process as integral asymmetric membranes where dense separation layer and porous support structure are made of the same material.
Furthermore, inorganic compounds both porous and non-porous with and without functional groups can be integrated into the membrane, e.g. aiming at the improvement of gas separation properties or membrane stability.
Model for describing the permeation behavior of gas separation membranes.
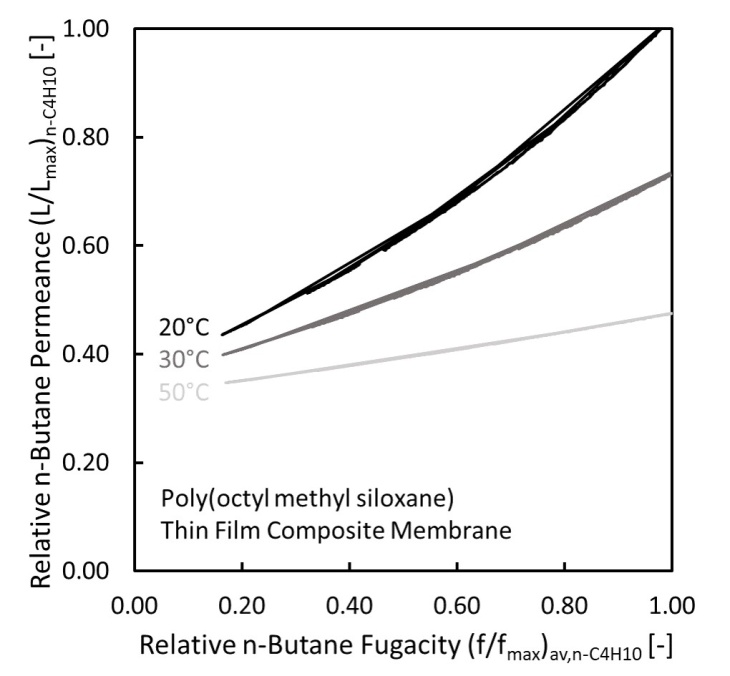
Image: Hereon/Torsten Brinkmann
The fundamental understanding of the effects contributing to the transport through membrane materials is essential to evaluate their operating performance. Automated apparatuses are employed for measuring the single gas permeation behaviour of membrane materials as a function of temperature and pressure.
The experimental data is described by means of permeation models. Gas mixtures are investigated using especially designed experimental setups. One target is the description of the multicomponent permeation behaviour by means of single gas permeation data and to validate this description by experiments.
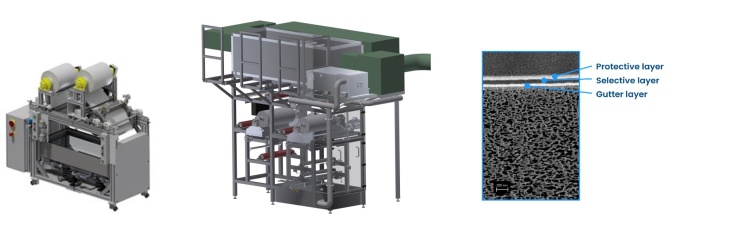
Images: Hereon/ Heiko Notzke
Flat sheet membranes are manufactured on a 100 m2 scale. The support structure for all membranes is prepared by the phase inversion principle. This results in porous sponge-like structures, which may have a dense film on the surface (integral asymmetric gas separation membrane). Most of membranes developed by Hereon are thin film composite, multilayered membranes. These are produced by coating the porous membranes prepared by the phase inversion process with one or more dense polymer layers. The thickness of these continuous, defect-free layers can be reduced down to 70 nm, which allows the production of high-flux membranes, as is required for many applications discussed today
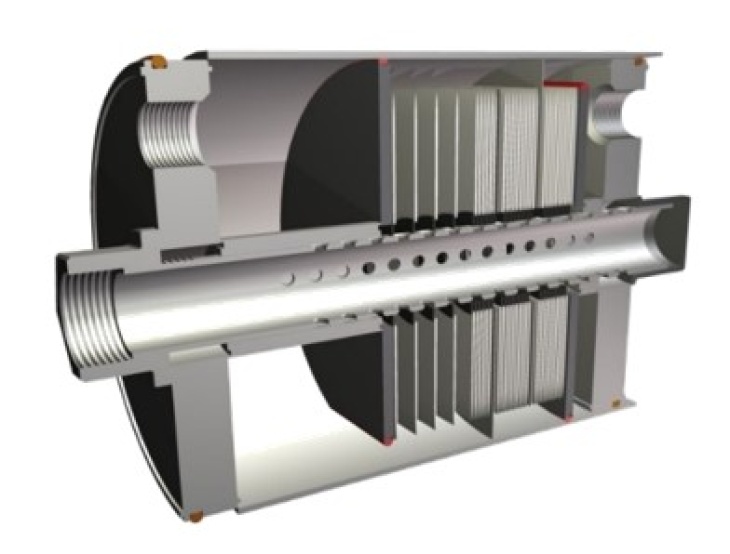
Image: Hereon/Thorsten Wolff
For transferring the intrinsic separation properties of a membrane material into the technical process of gas and vapour separation with only minimal losses in intrinsic membrane performance, process equipment has to be designed accordingly. The design of membrane modules aims at installing as much membrane area as possible per unit of module volume and at the same time to provide absence of negative effects during gas flow along and through the membrane surface. This include the minimisation of effects influencing the mass transfer through the membrane negatively, for example pressure losses and concentration polarization boundary layers.
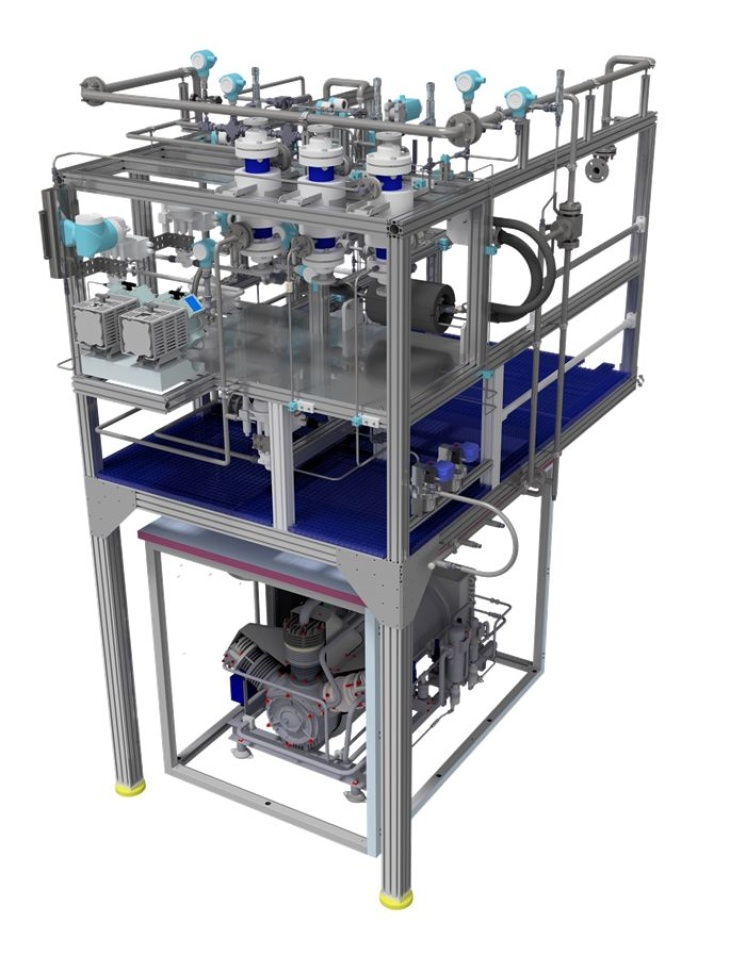
Image: Hereon/Heiko Notzke
The pilot plant infrastructure of the department is used to investigate the operating performance of newly developed membrane materials converted into membranes and installed in membrane modules under conditions closely resembling those of industrial applications. The feed mixtures are prepared in the lab or supplied by project partners.
Pilot plants are also operated at research partners sites where the feed streams are taken directly from the industrial process.
The investigations aim at the selection of the optimum process conditions with respect to pressure, temperature and composition ranges, the proof of long-term stability of the membrane material for a selected application, the evaluation of different membrane module concepts and the validation of simulation models.
Simulation models
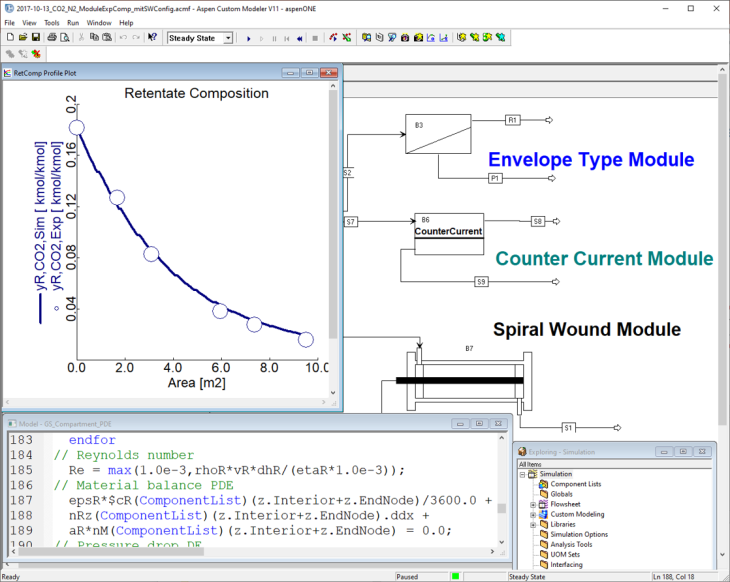
Image: Hereon/Torsten Brinkmann
For the design of membrane processes mathematical simulation models are employed. The simulation models describe the properties of the membrane modules as flow patterns, pressure distribution, velocity, concentration and temperature profiles and mass transfer through the membrane as a function of pressure, temperature and composition. Mathematically this leads to a system of ordinary differential and partial differential as well as algebraic equations.
These equations are implemented in appropriate software and the resulting models can be used in process simulators.
The process of membrane formation is described by a similar approach where a system of equations is developed to represent the formation of dense, selective layers on porous supporting structures. The consideration of different effects employing computational fluid dynamics methods is the basis of a knowledge-based optimization of the fabrication process.
In future, a further focus will be the description of the permeation behavior of multilayer thin film composite membranes. The subject is the assessment of the complex interaction of permeating molecule and polymer or matrix of polymer and inorganic compounds. Artificial intelligence methods will be employed to develop permeation models based on an extensive host of experimental results.
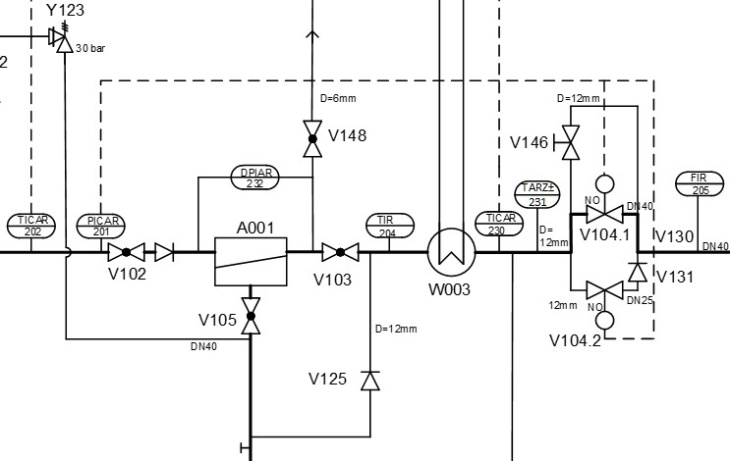
Image: Hereon/general
A membrane process does not only consist of the membrane module. The gas mixture supplied to the membrane plant has to be pretreated in order to remove potentially harmful components or condensates. Furthermore, the provision of the required driving force is essential in case it is not process inherent as e.g. in natural gas processing. For this, compressors and vacuum pumps or their combinations are employed.
Additionally, the permeation performance of membranes strongly depends on temperature: depending on the membrane material a temperature increase or decrease can affect separation process in terms of process selectivity and energy efficiency.
The proper integration of the membrane stage into the overall process model is also important: how does the membrane process interact with other reaction and separation stages and what parameter leads to a more efficeint process. For such an optimisation, process dynamics are of importance, e.g. for the development of the control strategy.
Process design answers these questions by a combination of pilot scale experiments, modelling and resulting designs. At the core is the experimentally validated mathematical model of the membrane module.