Application examples
Some examples from different institutes at the Helmholtz-Zentrum Hereon, which are currently running on the high-performance computer "Strand".
The department Hydrodynamics and Data Assimilation does numerical simulations of 3-D ocean circulation and ocean waves. The methods used are numerical modelling, data analysis and data assimilation. An emphathis lies on the data assimilation (for e.g. SST, SSS, Hs), which is the synergy between model and observations (in-situ, satellite, HF-radar) to provide a reliable products to end-users.
Another important activity is the integration of different models and the implementation of biogeochemical cycles in the ocean. In the integration of different models a coupling concept applies: interaction processes between wind and waves waves, so the atmosphere and ocean are calculated by separate models and exchange boundary informations through a coupler. This coupling enables the scientists to investigate non-linear feedbacks among the earth system components and are of special research interest.
All those tasks require a high amount of computing power and disk space to calculate. Here the HPC cluster "Strand" at the Helmholtz-Zentrum Hereon comes into focus and enables the scientists to do their research tasks efficiently.
Beside research the department provides support for operational system and running of pre-operational systems (COSYNA, BSH, DWD, German Navy, CMEMS).
Air quality has a high impact on health. Pollutants like NO2 or particulate matter affect the respiratory system of humans and animals and may lead to a decreased lifetime. In the department Chemistry Transport Modeling scientists take a closer look on air pollutants from various sources, their transport and chemical transformation in the air. Questions like 'What is the contribution of pollutant source X to the pollutant concentrations in region A?' or 'What if something would change (e.g. emissions, climate, policies, regulations)?' are answered by using numerical chemistry transport models.
The department Chemistry Transport Modellng at Helmholtz-Zentrum Hereon has a emphasis in the estimation and modeling of shipping emissions and the corresponding air concentrations in region scale. In various projects, scenarios with different legislations has been calculated.
Another key topic in the department is the city scale transport and chemistry modeling. As cities have very complex structures, air flow and dispersion of pollutants are less easy to predict than for the regional scale. In the context of shipping emissions and air pollution, harbor cities are of special research interest in the group. Beside the calculation of concentrations the department develops an emissions model named HiMEMO.
All those tasks consume a noticable amount of CPU time on HPC clusters and require massive data storage capacities. Here the cluster "Strand" provides the computing power and infrastructure required for these calculations.
Investigating the fundamental mechanisms of magnesium corrosion using atomistic simulations
Since experiments are not always sufficient to fully describe and understand the complex fundamental processes of magnesium corrosion, atomistic simulations offer an attractive possibility to provide deeper insights at the atomic level. The methods chosen are either based on empirical force fields as in classical molecular dynamics, or use ab initio methods such as density functional theory, which allows the direct calculation of the electronic structure. In this way it is possible to identify reaction barriers, describe charge shifts and generally gain an understanding of the energetic influences within the investigated reaction mechanisms. With the help of molecular dynamics, it is also possible to determine reaction kinetics and, for example, to analyze the degradation behavior of magnesium.
High-Performance-Computing (HPC) enables scientists of the department Atomistic Simulations the simulation of large systems (several hundred to several thousand atoms), as well as the implementation of high-throughput approaches for maximized data collection for machine learning applications. For example, energetically optimized molecular structures can be combined with experimental and atomistic simulation results to identify possible structure-property relationships.
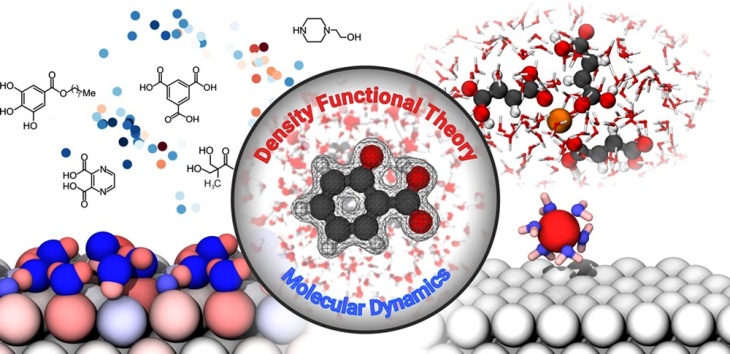
Methods for atomistic material modeling enable modeling of elementary corrosion processes on an atomic level. (from top left clockwise) Map of corrosion inhibitors based on molecular similarities; complexation of a magnesium ion by fumaric acid; a magnesium ion goes into solution; surface charges at the magnesium-water interface. (Image: Hereon)
Investigation of structure-property relations in nanoporous metals
Understanding the structure-property relationship of open-porous materials such as nanoporous gold requires detailed knowledge about the morphological and topological details of their microstructure. Established models like, e.g., the Gibson-Ashby scaling law which solely rely on comparatively easy to determine morphological parameters like the solid fraction turned out to be insufficient of capturing the macroscopic constitutive response of nanoporous metals. However, even with a detailed tomography of such microstructures at hand it is challenging to identify the relevant descriptors that adequately represent the 3D ligament network as input for a structure-property relationship.
In order to investigate the influence of the morphological and topological features such as ligament size, ligament shape and interconnection of the ligament network on the macroscopic constitutive response of nano-porous metals, systematic numerical studies with different discretization approaches (e.g. voxel or beam discretizations) of randomized diamond unit cells are carried out by scientists of the Institute of Materials Mechanics. As opposed to experiments, such numerical studies allow to systematically vary single microstructural features and thus help to isolate their influence on the macroscopic properties, ultimately revealing the microstructure descriptors based on which suitable structure-property relation can be derived.
Starting from perfectly ordered structures, the complexity can be subsequently increased by adding variations in ligament shapes, structural disorder, and connectivity. While approaching the morphology of real microstructures, it is possible to correlate each individual morphological parameter with its effect onto the macroscopic properties of the material in elastic, plastic and functional behavior. Based on this data we can obtain a clear picture about which descriptors are essential for the prediction of which effective properties.